What Is The Difference Between Gaussmeters And Teslameters?
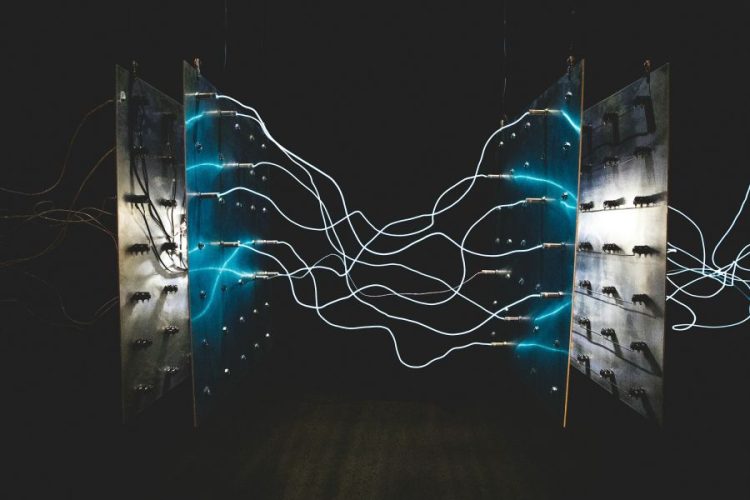
Categories :
In the realm of magnetism and electromagnetism, precise measurement tools are essential for understanding and manipulating magnetic fields. Gaussmeters and teslameters are two such devices that play a crucial role in measuring magnetic flux density. While they both serve similar purposes, there are distinct differences between them in terms of their design, units of measurement, applications, and accuracy. In this blog post, we'll explore these differences in detail to gain a better understanding of how gaussmeters and teslameters function and when to use each one.
Understanding Magnetic Field Measurement
Before we dive into the intricacies of gaussmeters and teslameters, it's crucial to have a solid grasp of the fundamental concept of magnetic field measurement. Magnetic flux density, represented as B, serves as a metric for quantifying the strength of a magnetic field. This measurement is typically expressed in units of tesla (T) or gauss (G), with the conversion ratio being 1 tesla equal to 10,000 gauss. These units are named after renowned physicists who have made substantial contributions to the realm of electromagnetism, further highlighting the significance and historical context behind these measurements. Whether you are looking for gaussmeters and teslameters like this or any other kinds, you should know that the concept of magnetic flux density is pivotal across numerous scientific and technological domains, ranging from electrical engineering and materials science to medical diagnostics and space exploration. By comprehending the essence of magnetic field measurement and its associated units, we can better appreciate the roles that gaussmeters and teslameters play in quantifying and analyzing magnetic phenomena with precision and accuracy.
Gaussmeters: Exploring Magnetic Field Strength in Gauss
Gaussmeters, aptly named for their measurement in gauss units, serve as indispensable tools for exploring and quantifying magnetic field strength. These instruments operate on the fundamental principle of the Hall effect, a phenomenon that occurs when a conductor experiences a perpendicular magnetic field concerning the current flow. This interaction results in the generation of a voltage difference across the conductor, known as the Hall voltage.
The Hall voltage is directly proportional to the strength of the magnetic field, allowing gaussmeters to precisely measure magnetic flux density in gauss units. This capability is particularly valuable in a wide array of applications, ranging from scientific research and engineering projects to industrial quality control and environmental monitoring.
By harnessing the principles of the Hall effect, gaussmeters provide researchers, engineers, and practitioners with valuable insights into magnetic field characteristics and variations. Whether in laboratory settings, manufacturing facilities, or field operations, gaussmeters play a pivotal role in advancing our understanding of magnetism and its myriad applications across diverse industries and scientific disciplines.
Design and Operation
Gaussmeters typically consist of a Hall effect sensor, a display unit (analog or digital), and associated circuitry for signal processing. The Hall sensor is positioned in the magnetic field to be measured, and as the field changes, the sensor generates a voltage proportional to the flux density. This voltage is then converted into gauss units and displayed on the instrument.
Units of Measurement
The primary unit of measurement for gaussmeters is gauss (G). They are particularly useful for applications where magnetic fields are relatively low, such as in laboratory experiments, electronics testing, and magnetic material characterization. Gaussmeters can detect subtle changes in magnetic flux density and provide precise readings in gauss increments.
Applications
Gaussmeters find extensive use in various industries and research fields. They are employed in magnet manufacturing to ensure the desired magnetic field strength, in medical devices like MRI machines for field calibration, and in environmental monitoring to assess electromagnetic pollution levels. Additionally, gaussmeters are valuable tools for educational purposes, allowing students and researchers to explore magnetic phenomena firsthand.
Accuracy and Limitations
The accuracy of gaussmeters depends on factors such as sensor quality, calibration, and environmental conditions. High-quality gaussmeters can achieve accuracies within a few gauss units, making them suitable for a wide range of applications. However, they may struggle to accurately measure extremely high magnetic fields, as the Hall effect sensor's response can saturate at certain levels.
Teslameters: Precision Measurements in Tesla
Teslameters, on the other hand, are instruments designed to measure magnetic flux density in tesla units. They operate on similar principles to gaussmeters but are optimized for higher magnetic field strengths and offer greater precision in tesla measurements.
Design and Operation
Teslameters employ various techniques for measuring magnetic fields, including Hall effect sensors, fluxgate sensors, and proton precession magnetometers. Fluxgate sensors, in particular, are commonly used in high-precision teslameters due to their ability to detect extremely weak magnetic fields with exceptional accuracy.
Units of Measurement
The standard unit of measurement for teslameters is tesla (T), named after Nikola Tesla, a pioneer in the field of electromagnetism. Teslameters are ideal for applications involving strong magnetic fields, such as in magnetic resonance imaging (MRI) machines, particle accelerators, magnetic levitation systems, and magnetic material testing for industrial purposes.
Applications
Teslameters play a critical role in industries where precise magnetic field measurements are paramount. In medical settings, teslameters are crucial for calibrating MRI machines to ensure accurate imaging results. They are also used in physics laboratories to study magnetic properties of materials and in aerospace applications for magnetometer calibration in spacecraft.
Accuracy and Limitations
Teslameters offer exceptional accuracy and sensitivity, often measuring magnetic fields down to microtesla (μT) or even nanoTesla (nT) levels. However, like gaussmeters, their performance can be influenced by factors such as sensor quality, calibration, and environmental conditions. Additionally, some teslameters may have limited range capabilities, requiring specialized models for extremely high magnetic field measurements.
Choosing the Right Instrument
When deciding between a gaussmeter and a teslameter, several factors must be considered:
Magnetic Field Strength: Gaussmeters are suitable for low to moderate magnetic field strengths, while teslameters excel in measuring high-strength fields.
Precision Requirements: If precise measurements in tesla units are necessary, a teslameter is preferred. For general magnetic field assessment in gauss units, a gaussmeter suffices.
Application Specificity: Consider the intended application and the range of magnetic field strengths involved. Select the instrument that best matches the requirements of the task at hand.
In conclusion, gaussmeters and teslameters are indispensable tools for measuring magnetic flux density in gauss and tesla units, respectively. While gaussmeters are adept at handling low to moderate magnetic fields with precision, teslameters excel in accurately measuring high-strength magnetic fields down to microtesla or even nanotesla levels. Understanding the differences between these instruments is crucial for selecting the right tool for specific applications across various industries, from electronics testing and material characterization to medical imaging and aerospace technology. By leveraging the capabilities of gaussmeters and teslameters effectively, researchers, engineers, and practitioners can harness the power of magnetism for diverse scientific and technological advancements.
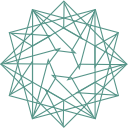
Citiesabc was created by a team of global industry leaders, academics and experts to create new solutions, resources, rankings and connections for the world’s top cities and populations.